Darryl D. D’ Lima, MD; Peter C. Chen, PhD; and Clifford W. Colwell, Jr., MD
Abstract:
Increased conformity at the tibiofemoral articulation increases contact area and reduces contact stresses in total knee arthroplasty. Malalignment, however, can increase polyethylene contact stresses. The effect of knee alignment and articular conformity on contact stresses was evaluated in a finite element model. The polyethylene insert and femoral component were modeled in highand low-conformity conditions. An axial tibial load of 3000 N was applied across the tibiofemoral articulation at different knee positions ranging from 00 to 900 flexion, 0 to 10 mm anteroposterior translation, 00 to 100 axial rotation, and coronal plane angulation (liftoff ). Increased conformity significantly reduced contact stresses in neutral alignment (by 44% at 00 flexion and 36% at 600 and 900 flexion). Liftoff significantly increased contact stresses in low- and high-conformity conditions, but to a lesser degree in the highconformity condition. Malalignment in rotation was most detrimental especially with the highconformity insert design. Overall, increasing articular conformity reduced stresses when the knee was well-aligned. However, malalignment in axial rotation was detrimental. Mobile-bearing knee designs with increased articular congruity may result in lower contact stresses, especially the rotating-bearing designs that theoretically minimize rotational malalignment.
From the Joint Mechanics Laboratory, Division of Orthopaedics, Scripps Clinic, CA.
Reprint requests to Clifford W. Colwell, Jr, MD, Scripps Clinic,
11025 North Torrey Pines Road, Suite 140, La Jolla, CA 92037.
Total knee arthroplasty is a successful surgical procedure with several reports of survivorship of more than 90% at as many as 15 years.3,12–14,18 However, because of the increase in life expectancy and the increase in the number of younger patients undergoing knee replacement, measures that additionally extend longevity must be evaluated. The knee is a complex articulation with nonlinear correlation between surface geometry, contact forces, and kinematics. Therefore, it often is difficult to predict the effect of design changes in variables that affect the above. Computer modeling and finite element analyses provide a means of testing the effect of individual variables such as change in prosthetic design and surgical techniques on knee forces and kinematics. This can offer valuable insights in knee biomechanics and also serve as an efficient screening tool before in vivo or even in vitro testing.
Fixed-bearing total condylar designs are low to moderately conforming to permit translations and axial rotations without significant increases in stresses. Increased conformity at the tibiofemoral articulation increases contact area and reduces contact stresses when the knee is well-aligned.5,11,19,20 However, articular malalignment either as a result of surgical technique or during activities of daily living can result in increased contact stresses. This may be even more detrimental in relatively high-conforming fixed-bearing designs. A finite element model that calculated contact area and contact stresses therefore was designed to study the interrelation of articular congruity and malalignment at different knee flexion angles.
Methods:
A three-dimensional finite element model of a knee prosthesis was generated in MARC (MSC software, Santa Ana, CA). The tibial insert was modeled as a deformable (flexible) body composed of 6700 eight-noded solid hexahedral elements (Fig 1). The insert was 8-mm thick and had an articular surface with a sagittal radius of 43 mm and a coronal radius of 22 mm. The femoral component was modeled as a rigid body. For the low-conformity condition, each femoral condyle had a distal sagittal articular radius of 33 mm, a posterior sagittal radius of 20 mm, and a coronal radius of 20 mm (Fig 2). For the high-conformity condition, each femoral condyle had a distal sagittal articular radius of 37 mm, a posterior sagittal radius of 25 mm, and a coronal radius of 21 mm. Nonlinear polyethylene material properties were obtained from experimental stress-strain and stress-relaxation data and were fit to a Mooney-Rivlin material model as reported previously.4,7
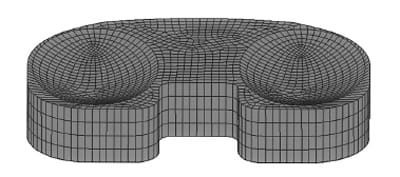
Fig 1. The insert was composed of 6700 eightnoded hexahedral elements with nonlinear material properties.
An axial tibial load of 3000 N (representative of three to four times body weight) was applied across the tibiofemoral articulation at different knee positions ranging from 00 to 900 flexion, 0 to 10 mm anteroposterior (AP) translation, 00 to 100 axial rotation, and 0 to 10 angulation in the coronal plane (liftoff ). Contact areas, contact stresses, and von Mises stresses were calculated for each conformity at each of the above mentioned positions.
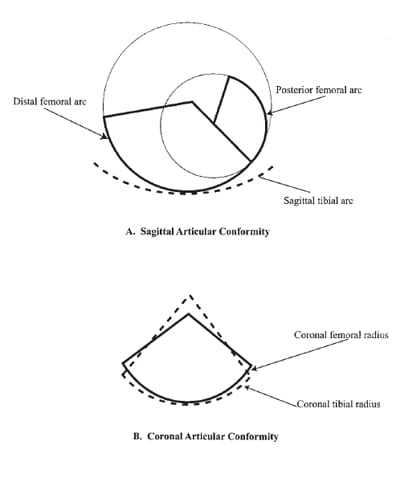
Fig 2A–B. Articular conformity between tibial and femoral surfaces is shown. (A) Sagittal conformity and (B) coronal conformity are shown.
To validate this model, a similar finite element model was created in MARC with manufacturer provided geometry and in vitro testing of a current generation knee arthroplasty design prosthesis. Using pressure-sensitive film (Fuji prescale film, Fuji Photo Film Co, Tokyo, Japan) and dye transfer method (Dykem, ITWDevcon, Irvine, CA) contact areas were measured at different loads in the prosthesis and compared with those predicted by the finite element model.
Results
The validation test showed that contact area measurements were consistently higher with the dye transfer method compared with the pressure-sensitive film measurements. The finite element model prediction tended to overestimate the contact area compared with that measured by pressure sensitive film, by approximately 10% to 12%. However, the relative differences at different loads were predicted more accurately
For all flexion angles, neutral alignment denotes well-centered femorotibial articular surfaces without
rotational or translational malalignment. Contact stresses at different alignment angles for knee flexion angles of 00 , 600 , and 900 are presented because these were taken to be representative of the heel strike portion of level gait, maximum flexion during stair climbing and rising from a chair, respectively (Table 1). Overall, von Mises stresses tended to correlate with contact stresses but were relatively lower. Neutral alignment at 00 flexion resulted in large contact areas in lowand high-conformity conditions. Knee flexion at 600 and 900 increased contact stresses by 51% as the (smaller) posterior sagittal radius of the femur came into play (Fig 3). Increased conformity significantly reduced peak contact stresses in neutral alignment (by 44% at 00 flexion and 36% at 600 and 900 flexion). Stresses at 600 and 900 flexion were comparable because in this range of knee flexion, the posterior femoral condyle (with the smaller radius) was in contact with the tibia
Liftoff (resulting in single condyle loading) significantly increased mean and peak polyethylene stresses in low- and high-conformity conditions. Again, high conformity stresses were much lower (by 26%) than low conformity in liftoff. Anteroposterior translations resulted in increased stresses for high- and low-conforming conditions (Table 1). The low-conformity condition translated 5 mm without significant increases in stresses whereas the high-conformity condition had higher stresses at 4 mm or more of posterior translation (Fig 4). Malalignment in rotation was the most detrimental especially with the high
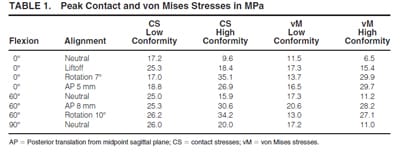
TABLE 1. Peak Contact and von Mises Stresses in MPa.
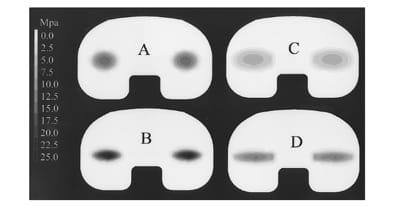
Fig 3A–D. Contour map of contact stresses for the two conformity conditions at neutral alignment are shown for (A) low conformity at 00 flexion, peak contact stresses are 17.2 MPa, (B) low conformity at 900 flexion, peak contact stresses increased by 51% (26.0 MPa), (C) high conformity at 00 flexion, peak contact stresses are 9.6 MPa, (D) high conformity at 900 flexion, peak contact stresses are 20.0 MPa.
conformity insert design. In full extension, stresses in the low-conformity design remained low up to 70 axial rotation. However, for the high-conformity condition, stresses began to rise at 30 axial rotation and crossed above the stresses for low conformity by 40 axial rotation (Figs 5, 6). The range of axial rotation without significant increase in stresses widened at higher flexion angles for both conditions, but the higher conformity consistently resulted in higher stresses than the low-conformity condition beyond 100 axial rotation at 600 and 900 knee flexion.
Discussion
The yield strengths of polyethylene materials in current use have been reported to range from 13 to 32 Mpa,1,2,6 with even lower values reported for the fatigue strength.21 Repeated
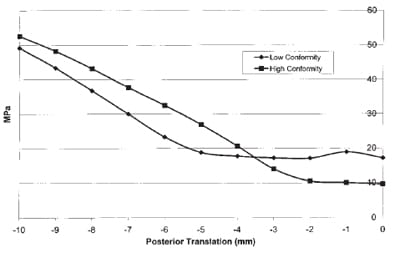
Fig 4. Peak contact stresses with posterior translation of the femur at 00 flexion under 3000 N axial load are shown. The high-conformity condition initially generates lower contact stresses that begin to rise above the low-conformity condition at 4 mm posterior translation.
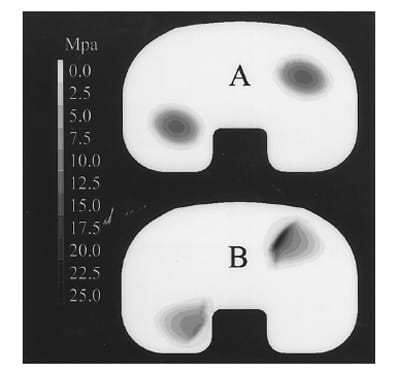
Fig 5A–B. Contour map of contact stresses with the femur rotated 50 at 00 flexion in (A) low-conformity design, and (B) high-conformity design. Peak contact stresses in the high-conformity design increased by 48%.
cyclic loading at stress levels approaching the yield strength may result in structural failure of the insert. Polyethylene wear also has been shown to correlate with contact stresses.15 Therefore, contact stress analysis of knee arthroplasty designs can be used to predict performance.
Several studies of contact stresses have been reported. Bartel et al1 reported on the beneficial effect of conformity and thickness on polyethylene contact stresses in a finite element model. They modeled prostheses commercially available at that time and therefore conformity and insert thickness varied between designs. Collier et al5 and Szivek et al19,20 measured contact stress distributions in several knee designs using pressure sensitive film. Larger contact areas and lower stresses were reported with higher conforming designs. The above reports were for well-aligned prostheses. Sathasivam and Walker 16,17 used a combination of rigid body analysis to predict tibiofemoral position and finite element analysis to calculate contact stresses under load. The rigid body analysis used forces derived from gait analysis data for level walking in healthy patients to determine the tibiofemoral relative position and orientation during the stance phase. Polyethylene contact stresses and subsurface stresses then were computed at each of these positions for models with changes in design parameters. The authors predicted the least susceptibility to delamination in the design with the larger femoral frontal radius and higher conformity. No attempt at malalignment was made in these studies.
Matsuda et al11 measured contact stresses in fixed- and mobile-bearing prostheses. They reported higher stresses with the fixed-bearing compared with the meniscal bearings at neutral alignment and at 150 rotational malalignment. Harman et al9 compared knee kinematics obtained by in vivo fluoroscopy with retrieved inserts from the same patients. The in vivo tibiofemoral contact location in the AP direction and in rotation was found to correlate well with the wear patterns seen on the tibial inserts. Hood et al10 did a clinical retrieval analysis and reported that as many as 20% of retrievals were associated with radiographic malpositioning of the knee prosthesis. Together, these reports strongly suggest that articular conformity and knee position can be correlated with contact stresses and wear. A systematic study of the effect of tibiofemoral articular conformity at different knee positions and alignments would be useful in providing insights in the interrelation between these variables. Therefore, a finite element model was developed to study this effect.
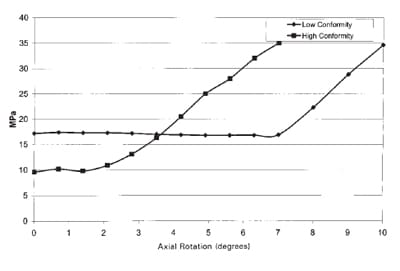
Fig 6. Peak contact stresses with axial rotation of t
he femur at 00 flexion under 3000 N axial load show that the high-conformity condition initially generates lower contact stresses that begin to rise above the low-conformity condition at 3.50 axial rotation.
In neutral alignment at 00 flexion, low- and high-conformity conditions resulted in large contact areas. Knee flexion reduces contact area and increases contact stresses as the smaller posterior sagittal radius of the femur articulates with the tibia. Increasing conformity significantly reduced stresses when the knee was wellaligned. This is consistent with experimental data previously reported.1,5,11,19,20 In neutral alignment and during liftoff, the high-conformity condition tended to reduce stresses relative to the low-conformity design. Anteroposterior translation resulted in higher stresses in the high-conformity condition after 4 mm translation. Malrotation caused the greatest increase in stresses especially in the high-conformity design and seemed to be the most detrimental. However, knee flexion allowed for an increase in the range of axial rotation before stresses were significant affected.
Fishkin et al8 compared contact areas and rotational constraint between an implant with dual radius condylar geometry and a design with one mediolateral radius for both condyles. They reported significantly higher torsional stiffness in the dual radius design, which is consistent with the current findings. The current authors, however, did not study the effect of one radius design. The findings that high articular conformity designs reduce stresses but are relatively intolerant of rotational malalignment support the use of higher conformity mobile-bearing design prostheses especially rotating platform designs that potentially can self-align in the presence of malrotation. This also has been borne out in an experimental contact stress analysis of meniscal bearings.11
The high-conformity design parameters tested resulted in lower stresses than the lowconformity design in neutral alignment and liftoff. Anteroposterior translation seemed to increase stresses to a moderate degree in the high conformity relative to the low conformity. Rotational malalignment increased stresses the highest in the high-conformity design. The current authors examined a rather narrow set of design parameters. However, this model provides an efficient means of systematically analyzing a wider range of design features.
REFERENCES
- [1]Bartel DL, Rawlinson JJ, Burstein AH, et al: Stresses in polyethylene components of contemporary total knee replacements. Clin Orthop 317:76–82, 1995.
- [2] Buechel FF, Pappas MJ, Makris G: Evaluation of contact stress in metal-backed patellar replacements: A predictor of survivorship. Clin Orthop 273:190–197, 1991.
- [3] Buehler KO, Venn-Watson E, D’Lima DD, et al: The press-fit condylar total knee system: 8- to 10-year results with a posterior cruciate-retaining design. J Arthroplasty 15:698–701, 2000.
- [4] Chen PC, Pinto JG, D’Lima DD, et al: Polyethylene material properties: Stress relaxation. World Biomater Cong 6:1469, 2000. Abstract.
- [5] Collier JP, Mayor MB, McNamara JL, et al: Analysis of the failure of 122 polyethylene inserts from uncemented tibial knee components. Clin Orthop 273:232–242, 1991.
- [6] Collier JP, Mayor MB, Surprenant VA, et al: The biomechanical problems of polyethylene as a bearing surface. Clin Orthop 261:107–113, 1990.
- [7] D’Lima DD, Chen PC, Pinto JG, et al: Finite element model of UHMWPE. World Biomater Cong 6:133, 2000. Abstract.
- [8] Fishkin Z, Serpe L, Kester MA, et al: Maximizing conformity without excessive rotational constraint in TKA. Trans Orthop Res Soc 24:958, 1999.
- [9] Harman MK, Banks SA, Natarajan RN, et al: Comparison of in-vivo kinematics and polyethylene wear in retrieved total knee replacements. Trans Orthop Res Soc 23:368, 1998.
- [10] Hood RW, Wright TM, Burstein AH: Retrieval analysis of total knee prostheses: A method and its application to 48 total condylar prostheses. J Biomed Mater Res 17:829–842, 1983.
- [11] Matsuda S, White SE, Williams VG, et al: Contact stress analysis in meniscal bearing total knee arthroplasty. J Arthroplasty 13:699–706, 1998.
- [12] Ranawat CS, Boachie-Adjei O: Survivorship analysis and results of total condylar knee arthroplasty: Eight- to 11-year follow-up period. Clin Orthop 226:6–13, 1988.
- [13] Ranawat CS, Flynn WFJ, Deshmukh RG: Impact of modern technique on long-term results of total condylar knee arthroplasty. Clin Orthop 309:131–135, 1994.
- [14] Ranawat CS, Flynn WFJ, Saddler S, et al: Long-term results of the total condylar knee arthroplasty: A 15- year survivorship study. Clin Orthop 286:94–102, 1993.
- [15] Rostoker W, Galante JO: Contact pressure dependence of wear rates of ultra high molecular weight polyethylene. J Biomed Mater Res 13:957–964, 1979.
- [16] Sathasivam S, Walker PS: Computer model to predict subsurface damage in tibial inserts of total knees. J Orthop Res 16:564–571, 1998.
- [17] Sathasivam S, Walker PS: The conflicting requirements of laxity and conformity in total knee replacement. J Biomech 32:239–247, 1999.
- [18] Scuderi GR, Insall JN: Total knee arthroplasty: Current clinical perspectives. Clin Orthop 276:26–32, 1992.
- [19] Szivek JA, Anderson PL, Benjamin JB: Average and peak contact stress distribution evaluation of total knee arthroplasties. J Arthroplasty 11:952–963, 1996.
- [20] Szivek JA, Cutignola L, Volz RG: Tibiofemoral contact stress and stress distribution evaluation of total knee arthroplasties. J Arthroplasty 10:480–491, 1995.
- [21] Weightman B, Light D: A comparison of RCH 1000 and Hi Fax 1900 ultrahigh molecular weight polyethylenes. Biomaterials 6:177–183, 1985.