Abd Rahim Abu Bakar and Huajiang Ouyang – Department of Engineering, University of Liverpool
Jennifer E. Siegel – Sensor Products LLC, USA
ABSTRACT
Recent studies carried out at the University of Liverpool showed that there was a variation in static contact pressure distributions on two new sets of pads even though they were tested with the same brake system under the same pressure. One of the possible reasons for this variation is due to brake pad surface topography.
This paper investigates the effects of brake pad topography on contact pressure distributions. Pressurex®, pressure indicating films are used to measure static contact pressure distribution. A detailed 3-D finite element model of a disc brake is also developed and takes into account pad surface profiles. A linear gauge is used to measure brake pad topography. Six different pad configurations are studied in this investigation. Results from the investigation could provide a better explanation of the variation in contact pressure distribution and in turn squeal generation.
INTRODUCTION
Research on disc brake interface pressure distributions has been carried out by a number of researchers either experimentally or numerically. Tumbrink [1] is the first researcher who measured disc brake contact pressure distributions and used a ball pressure method. Later, Samie and Sheridan [2] conducted static contact pressure tests using Pressurex® sensor film and compared the results to their 3-D finite element (FE) model. They commented that good correlation was obtained for both piston and finger pads, qualitatively and quantitatively. However, they did not mention whether or not brake pads surface topography was included in their FE model. Contact pressure distribution was studied numerically using a detail 3-D finite element model in [3- 5]. Despite increasing details in their FE models, none of them considered the brake pad surface topography.
There has not been much research on the variation of contact pressure distribution for different pairs of pads. In a recent review, Chen et al [6] showed that there appeared noticeable variations in static contact pressure distribution between two new sets of pads, even though the pads have the same specifications and were tested with the same brake system under the same pressure. The reason for these variations was not given. Recent works by the authors at the University of Liverpool showed that such a variation indeed existed and was significant. The authors believe that one of the possible reasons for the variation is due to brake pad surface topography and attempt to demonstrate this in this paper. The surface profiles for each pairs of pads are not the same and thus might generate different contact pressure distributions.
Therefore, the motivation of this paper is to investigate the effects of brake pad topography on contact pressure distributions first experimentally and then numerically. In doing so, a specific type of pressure-indicating films that has a certain pressure range is used. The tested films were then analyzed using Topaq® Pressure Analysis System in order to obtain both magnitude and distribution of the contact pressure. Six different pad configurations are studied in this investigation. These include a pair of worn pads, three new sets of pads with and without the damping shims, and applied under different levels of brake-line pressure. The effects of damping shims and brake-line pressure are also studied. In order to complete the investigation, a detail 3-dimensional finite element model of a disc brake is also developed. Brake pads are modelled that takes into account surface profiles. A Mitutoyo linear gauge is used to measure brake pad surface topography. A comparison in contact pressure distributions and its magnitude will be made between simulated and tested results.
CONTACT PRESSURE TESTS
The contact pressure tests are conducted using an inhouse disc brake dynamometer. The solid disc brake system of floating caliper design being investigated is shown in Fig. 1.
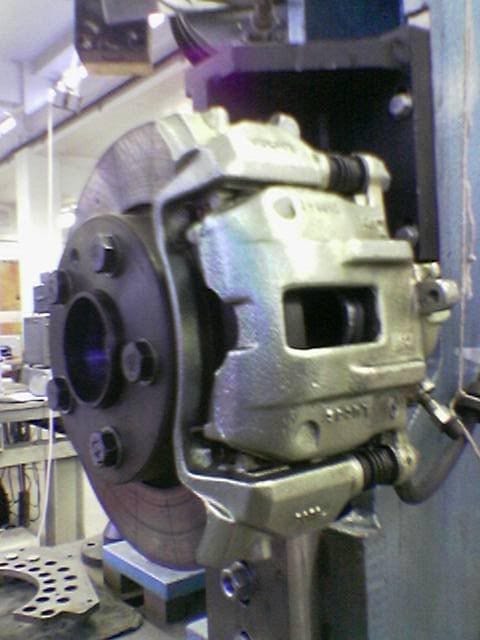
Figure 1. A solid disc brake system
PRESSUREX® PRESSURE INDICATING FILM
In order to measure contact pressure distributions, a suitable type of sensor film should be chosen for a particular range of local contact pressure. In this work, Pressurex® Super Low (SL) pressure-indicating film, which can accommodate contact pressure in the range of 0.5 ~ 2.8 MPa is selected. The film needs to be cut to the shape of the brake pad for it to be well positioned in the pad/disc interface. Brake-line pressure at certain levels is then applied to the disc brake for 30 seconds and then removed. Figure 2 shows films before and after brake pad testing.
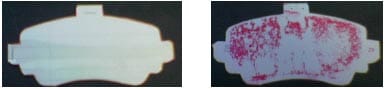
Figure 2. Pressurex® Super Low pressure indicating film before (left) and after (right) testing.
TOPAQ® PRESSURE ANALYSIS SYSTEM
It is not quite sufficient to describe contact pressure distributions by showing only the stress marks on the tested films. The contact pressure distributions should be measured qualitatively and quantitatively for comprehensive understanding. In doing so, a system called Topaq® Pressure Analysis system is used. The Topaq® system is a post-process interpretive system that analyzing pressure distribution and magnitude from a proprietary tactile transducer or commonly known as pressure indicating film. The system consists of calibrated densitimetric scanner and Windows software. The system is used to image and interpret the stress marks on the tested film. The system, offered by Sensor Products LLC as a product for purchase or as a service, is accurate to within 4% – unprecedented in the field of tactile pressure measurement.
BRAKE PAD SURFACE TOPOGRAPHY
A linear gauge LG-1030E from Mitutoyo is used to measure the brake pad surface topography. An arrangement of brake pad measurement is shown in Fig. 3. The measurements are taken at specific locations that are provided by finite element nodes.
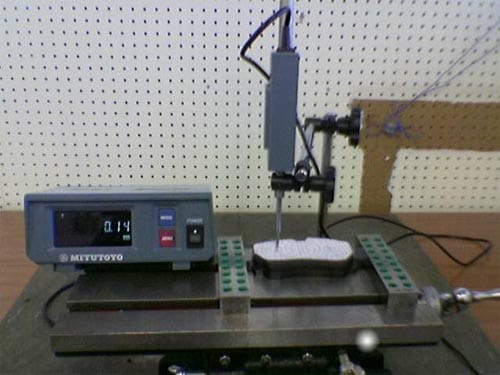
Figure 3. An arrangement of brake pad surface measurements.
The data thus obtained can describe roughly the topography of the tested pad surface. Figure 4 shows surface topographies for different pairs of the pads.
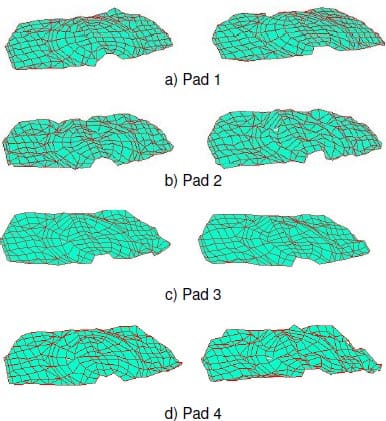
Figure 4. Brake pad surface topography
From the illustrations in Fig. 4, it is clear that each pad has a different surface topography despite being produced by the same manufacturer and interestingly, even though they come from the same box (Pad 3 and 4). This indicates that when the pairs of pads are fitted in the brake system, it may produce different contact pressure distributions and consequently may generate different braking torque both at right and left wheels of a vehicle. In addition, generation of squeal noise may also differ at both wheels. It is also shown that Pad 1, i.e., the worn pads have smoother surface than the new sets of pads. The surface topographies are also different for the piston and the finger pads. This is due to the way force is applied to brake backing plates.
The difference in surface topography for the new sets of pad seems to be a manufacturing process defect. The variation in surface topography could result in difference in contact pressure distributions. This is discussed in the next section.
CONFIGURATIONS OF BRAKE PAD
The investigation is not only to obtain the effects of brake pad surface topography but also to study the effects of damping shim and the effects of brake-line pressure on static contact pressure distributions. Thus, four different pads with six different configurations are tested and they are described in Table 1. It is worthy to mention that the same manufacturer produced all the pads. Therefore, the authors assume that they have the same specifications.
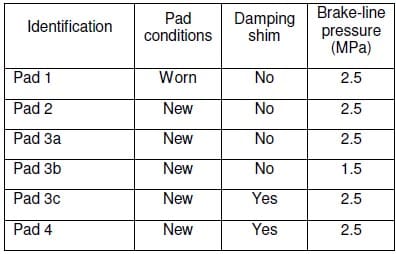
Table 1. Configurations of tested pad
Figure 5 shows results on contact pressure distributions that have been analysed using the Topaq® system. It clearly shows that the variation in surface topography could produce different contact pressure distributions. Figure 3c and 4 show static contact pressure distributions of the pads from the same box. Similarly, there are differences between Pad 2 and Pad 3a, which are from separate boxes. By looking at those results, it can be said that variations in contact pressure distributions are due to brake pad surface topography. This, in turn could also explain the variations in static contact pressure distributions found in [6].
A comparison is also made between the pads with and without the damping shim. Figure 3a and 3c show that there is a little difference between them. The areas of the highest contact pressures (shown in red in the interpreted images of Fig.3) of the pads with the damping shim at certain locations seem to be less than those of the pads without the damping shim. This may be due to an extra thickness provided by the damping shim at the piston and finger pads.
By applying different levels of brake-line pressure, the higher the pressure the more the contact areas generated and the higher the maximum contact pressure. This is understandable and the results prove it. While for the worn pad, the highest contact pressure seems to be at one specific area compared to the new pads. In general, there appears zero pressure at the trailing edge of the pads and most of the higher contact pressures are present at the outer border region of the pads.
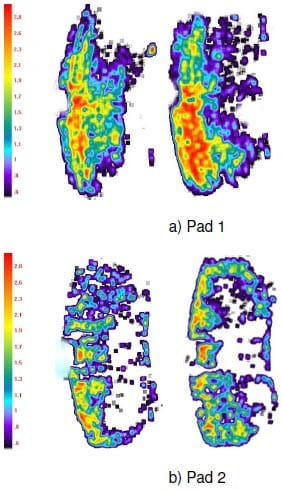
Figure 5. Analysed images of the tested pads: piston pad (left) and finger pad (right). Pressure is measured in MPa.
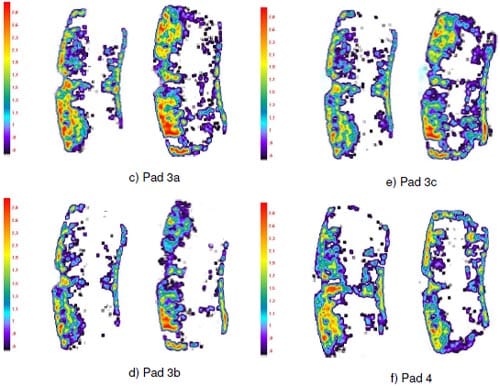
Figure 5 (cont’d). Analysed images of the tested pads: piston pad (left) and finger pad (right). Pressure is measured in MPa.
FINITE ELEMENT MODEL
The finite element (FE) model, as shown in Fig. 6, consists of a disc (rotor), two pads, a caliper, a carrier, a piston and two guide pins. Damping shims are not present in the FE model. The model uses up to 8000 solid elements and approximately 37,200 degrees of freedom.
In the past, most researchers attempted to simulate dynamic contact pressure distributions using numerical methods since there are no experimental methods available. However, measuring static contact pressure distributions is still possible and there are methods available to do so. This, to the authors, could help researchers to gain more confidence as they can use experimental results on contact pressure distributions to validate their models. In the brake research community such validation has not been attempted. In the authors’ point of view, this also could provide better prediction in squeal generation where the complex eigenvalue analysis strongly depends on the inclusion of stiffness matrix that is derived from the contact pressure distributions.
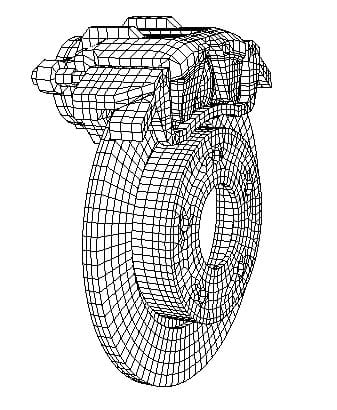
Figure 6. Finite element model of a disc brake.
In this section, simulation of contact analysis is performed having considered the brake pad surface topography, i.e., by adjusting co-ordinates of finite element nodes on the surface of the pads. Brake surface topographies of the pads being simulated are shown in Fig. 4(a) – 4(c).
Figure 7 shows results of static contact pressure distributions that assume that the pads have smooth and flat surfaces. The predicted results seem to show that the contact pressure distributions are symmetrical for both the piston and finger pads whereas the analyzed images show differently.
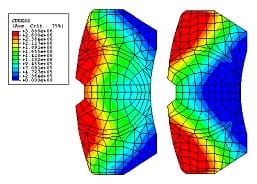
Figure 7. Contact pressure distributions of smooth and flat pads.
Whilst from Fig. 8 that considers brake pad surface topography, the simulated contact pressure distributions seem to provide more realistic results qualitatively and quantitatively. Good correlations are achieved between the simulated and tested results for those three pads. This suggests that validation through the contact analysis can be done and implemented especially when one’s attempt to investigate squeal noise prediction using the finite element method. The investigation on squeal noise generation where the FE model has been validated through the contact analysis is discussed in [7].
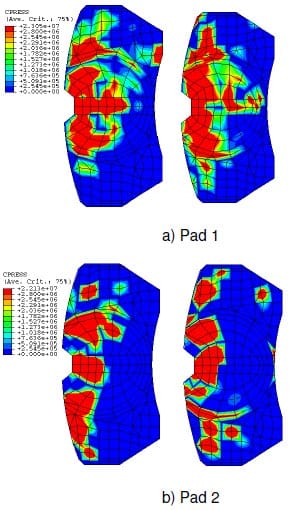
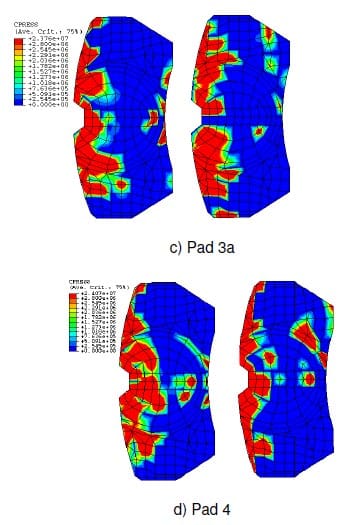
Figure 8. Simulation results of the tested pads: piston pad (left) and finger pad (right).
CONCLUSIONS
This paper reveals that one of the reasons for variation in the contact pressure distribution is due to the brake pad surface topography. Measurements at the surface of the pads, using the linear gauge, were clearly shown that the pads have irregular and corrugated surface. Thus, an assumption that the pads have flat and smooth surface that has been adopted in the FE model is no longer valid. The simulated results using such an assumption indicated that there were no good correlations to the tests results whereas the FE model that considered pad surface topographies provides more realistic results and good correlations were achieved.
There is a little difference in the contact pressure for pads with and without damping shims. The test results also proved that the change in brake-line pressure results in different contact pressure distributions, where the higher the brake-line pressure the higher the maximum contact pressure and vice-versa. The worn pads seems to produce more concentrated contact pressure than the new sets of pads, where most of the pressure is present a
t the outer border region of brake pads.
Finally, this paper suggests that in order to predict squeal noise generation, an FE model should be validated through static contact pressure distributions, which can and should be done. The Pressurex® pressure indicating film and Topaq®. system are useful as a validation tools.
ACKNOWLEDGMENTS
The main author wishes to thank the Government of Malaysia and Universiti Teknologi Malaysia for sponsoring the study and Sensor Products LLC for donating Pressurex® sensor film and the Topaq® pressure analysis system.
REFERENCES
- Tumbrink, H. J. “ Measurement of load distribution on disc brake pads and optimization of disc brake using the ball pressure methods”. SAE Paper 890863, 1989.
- Samie, F. and Sheridan, D. C. “Contact analysis for a passenger cars disc brake”. SAE Paper 900005, 1990.
- Tirovic, M. and Day, A. J. “ Disc brake interface pressure distributions”. Proc. IMechE, Part D, 205, pp. 137-146, 1991.
- Hohmann, C., Schiffner, K., Oerter, K. and Reese, H. “ Contact analysis for drum brakes and disk brakes using ADINA”. Computers and Structures, 72, pp. 185-198, 1999.
- Abu Bakar, A. R. and Ouyang, H. “Contact pressure distributions by simulated structural modifications”. Proc. of the Int. Conf. on Braking 2004 – Vehicle Braking and Chassis Control, pp. 123- 132, 2004.
- Chen, F., Abdelhamid, M. K., Blaschke, P. and Swayze, J. “On automotive disc brake squeal PartIII: Test and evaluation”. SAE Paper 2003-01-1622, 2003.
- Abu Bakar, A. R., Ouyang, H. and Siegel, J. E. “ Brake pad surface topography Part II: Squeal generation and prevention”. SAE Technical Paper, 2005-01-3935,2005.