Christopher S. Ahmad,*† MD, Maxwell C. Park,† MD, and Neal S. ElAttrache,‡ MD
From the †Center for Shoulder, Elbow, and Sports Medicine, Department of Orthopaedic Surgery, Columbia University, New York, New York, and the ‡Kerlan-Jobe Orthopaedic Clinic, Los Angeles, California
Background
The effect of elbow medial ulnar collateral ligament injury on posteromedial compartment contact is unknown.
Hypothesis
Medial ulnar collateral ligament injury causes altered contact area and pressure in the posteromedial compartment of the elbow.
Study Design
Controlled laboratory study.
Methods
Seven elbow cadaveric specimens were tested in an apparatus that positioned the elbow at 30° and 90° of flexion. Partial and full tears were simulated by releasing the medial ulnar collateral ligament. Pressure-sensitive film was placed in the posteromedial compartment for each condition. Valgus torques of 1.25 and 2.0 N.m were applied for each ligament condition, and kinematic data were obtained at each flexion angle using a 3-dimensional digitizer.
Results
Both ligament condition and valgus load had significant effects on contact area and pressure (P < .05). For a given load and flexion angle, the contact area decreased and the pressure increased with increasing medial ulnar collateral ligament insufficiency. Within these trends, statistical significance was found at 30° of elbow flexion for both area and pressure (P < .05); at 90° of elbow flexion, increasing medial ulnar collateral ligament insufficiency did not significantly affect contact area or pressure (P > .05).
Discussion
Medial ulnar collateral ligament insufficiency alters contact area and pressure between the posteromedial trochlea and olecranon and helps explain the development of posteromedial osteophytes.
Keywords – elbow; collateral ligament; biomechanics
During overhead throwing, tremendous valgus forces are generated across the elbow during the acceleration phase of the throw, which results in extreme tensile forces on the medial side of the elbow.17,26 The medial ulnar collateral ligament (MUCL) is the primary valgus stabilizer to the flexed elbow,21-23 and repeated stresses may cause attenuation or rupture of the MUCL.5,17 After the acceleration phase of throwing, established arm momentum causes an elbow extension force that is resisted by the flexor muscles of the elbow.13,14 If deceleration is not controlled dynamically by the elbow flexor muscles as the elbow extends, the olecranon may abut the posteromedial compartment.
Repetitive compression injuries in the posteromedial compartment cause posteromedial olecranon osteophytosis, resulting in pain and limited extension.5,8 Treatment of these extension overload injuries has included conservative management, arthroscopic debridement, and open resection of the posteromedial osteophytes.3,5,33
Andrews et al reported on a series of competitive baseball players who underwent isolated resection of symptomatic posteromedial osteophytes and found that 25% developed valgus instability and eventually required MUCL reconstruction.5 Conway et al reported that more than 50% of their patients undergoing MUCL repair or reconstruction had posteromedial osteophytes.10 These studies highlight a relationship between the MUCL and the posteromedial olecranon and suggest that both the olecranon and the MUCL contribute to valgus stability.
However, the direct effects of MUCL injury on posteromedial compartment articular contact have not been established. We hypothesize that MUCL injury resulting in valgus instability causes abnormal contact area and pressure within the posteromedial compartment of the elbow. The objective of this study was to characterize the effects of MUCL injury on olecranon contact within the trochlea in a cadaveric model.
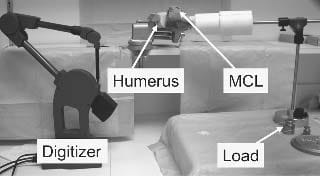
Figure 1. Digitizer and experimental testing apparatus with radius and ulna constrained only to flexion. MCL, medial collateral ligament.
Methods
Seven adult (3 female, 4 male) elbow fresh-frozen cadaveric specimens were dissected free of soft tissues except for the capsule and ligaments. Although the ages of the specimens were unknown, none of the elbows showed evidence of articular degenerative change. The humerus, radius, and ulna were osteotomized 14 cm from the joint line and rigidly fixed within polyvinyl chloride (PVC) pipe with plaster. The radius and ulna were positioned in neutral pronation supination, as in numerous other studies.2,4,9,11,29
The PVC pipe holding the humerus was held fixed in a vise grip. A smooth steel rod was attached to the forearm and PVC pipe. A positioning device held the steel rod, maintaining the elbow at the desired flexion angles of 30° and 90° (Figure 1). A gravity valgus position was used, placing the humerus horizontal to the floor, with the radius inferior and the ulna superior.24 Flexion-extension was the only constraint to motion; the elbow was allowed to move freely into valgus and rotate along an axis in line with the ulna.
Pressure-sensitive film (Prescale ultra super low pressure type, Fuji photo film, C. Itoh & Co Inc, New York, NY) was used to measure the interface contact area and pressure between the posteromedial trochlea and olecranon, similar to other studies of the elbow.20,30 The pressuresensitive film was shaped to conform to the curvature of the trochlea and cut in a standardized fashion for all specimens (Figure 2).
Valgus torques of 1.25 and 2.0 N.m, approximating 3% and then 6% of maximum failure torque, were applied across the elbow, similar to studies by Sojbjerg et al (1.5 N.m)29 and Andrews et al (1.90 Nxm).4 The PVC pipe and steel rod assembly, with its inherent weight, created the 1.25-N.m moment across the elbow. A hanging 250-g weight was applied to increase the torque to 2.0 N.m.
For each elbow specimen per test, each contoured piece of film was placed in the same orientation in an identical location on the trochlea within the posteromedial elbow (Figure 3). During placement of the film, the joint was distracted, and shear to the film was avoided. The pressuresensitive film was loaded for 30 seconds per test. During the loading of each elbow, 3 constant points in space along the ulna, relative to 3 constant points along the humerus, were digitized to obtain valgus angles using a 3-dimensional coor
dinate measuring machine (Microscribe model, Immersion Corp, San Jose, Calif). The accuracy of this device has been determined to be 0.30 mm.27 The valgus angle was calculated from the digitized points at each flexion angle tested. This is consistent with numerous studies that have used valgus angle measurements to objectively and reproducibly assess valgus stability.1,19,22,24
After loading, each piece of film was scanned (Epson Expression 363, Epson America, Inc, Torrance, Calif) immediately to create a digital image alongside both length and color-intensity scales using a personal computer. National Institutes of Health Image software (Scion Image Beta 4.0.2, Scion Corporation, Frederick, Md) was used to analyze the digital image in grayscale. The colorintensity scales provided with the film were used to calibrate the image software, a technique similar to those previously reported.28 The contact area on the pressurized film images was manually digitized using the image software, with the digitizer blinded to the testing condition. The image software, which computes area and pressure according to the calibration, was then used to analyze the experimental film images to determine the contact area and pressure.
Partial MUCL injury was simulated by sharply recessing the deepest fibers of the anterior bundle from the humeral attachment at the medial epicondyle without completely detaching the ligament, until 1 mm of joint space opening was observed at 30° of elbow flexion. All pressure-sensitive film and valgus angle measurements were made at both flexion angles, first with 1.25 N.m of torque and then with 2.0 N.m of torque applied. The loads chosen were safely below the torques to failure reported in the literature.1,16 Complete MUCL injury was then achieved by completely releasing the deep fibers of the anterior bundle from its humeral attachment, which in all cases demonstrated greater than 2 mm of joint space opening at 30° of elbow flexion. The majority of complete MUCL tears in overhead-throwing athletes have been reported to occur proximally (68%).7
Each elbow underwent pressure-sensitive film and valgus angle data acquisition for 3 ligament conditions: intact, partial tear, and full tear. For each condition, data were obtained at 30° and 90° of elbow flexion. For each ligament condition and elbow flexion angle, data were obtained first with 1.25-N.m loads and then with 2.0-N.m loads.
Statistical Methods
All testing conditions were tested in the same specimen. Statistical analysis was performed using a 3-factor analysis of variance with repeated measures for all factors. The 3 factors evaluated were elbow condition (intact, partial MUCL tear, complete MUCL tear), flexion angle, and load. When significant differences were found (P < .05) using a 3-way analysis of variance, a Student-Newman-Keuls multiple comparisons test was used to isolate the specific significant differences.
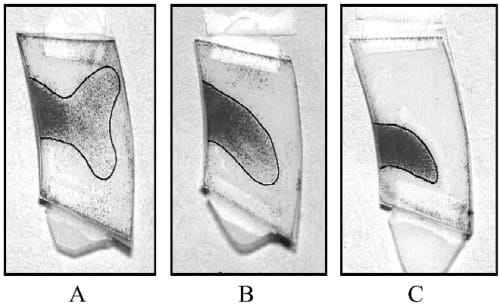
Figure 2. Typical pressure-sensitive film for (A) intact, (B) partial, and (C) complete medial ulnar collateral ligament tears after application of a 2.0-N.m torque. The bottom of each contoured piece of film represents the distal aspect of the posteromedial trochlea; the right edge represents the medial aspect. The area, as defined by the dark lines, grossly decreases from A to B to C; the density of color, as a measure of pressure, increases.
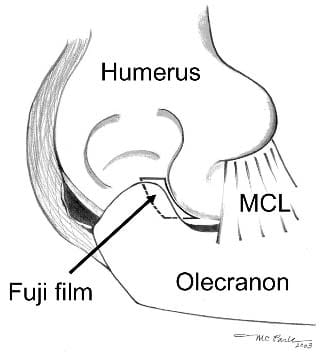
Figure 3. Pressure-sensitive film placed on the posteromedial trochlea of the elbow. MCL, medial collateral ligament.
Results
Figure 2 displays representative examples of pressuresensitive film after testing with a 2.0-N.m load at 30° of elbow flexion. The bottom of each custom-cut piece of film represents the distal aspect of the posteromedial trochlea; the right side of each piece represents the most medial aspect. The area decreases with increasing MUCL insufficiency from A to B to C; the density of color that indicates pressure magnitude increases.
For all ligament and loading conditions, the contact area ranged from 20.1 to 43.2 mm2. For a given load and flexion angle, the average contact area decreased with increasing MUCL insufficiency (Table 1). A significant decrease in contact area was observed for the full tear condition at 30° of elbow flexion against a 2.0-N.m load when compared to the intact and partial MUCL tear conditions (P < .05).
For all ligament and loading conditions, the contact pressure ranged from 0.37 to 0.93 MPa. For a given load and flexion angle, the average contact pressure increased with increasing MUCL insufficiency (Table 2). The full tear conditions for both loads, at 30° of elbow flexion, were found to have significantly increased pressures compared to the intact and partial MUCL tear conditions (P < .05).

TABLE 1 – Average Contact Areas With Standard Deviations (mm2)

TABLE 2 – Average Contact Pressures With Standard Deviations (MPa)

TABLE 3 – Average Valgus Angles Relative to the Intact MUCL Condition (degrees)a
Table 3 displays the results of changes in valgus angle, relative to the intact MUCL valgus angle, with load condition at each elbow flexion angle. Full tears showed statistically significant changes with a maximum valgus opening of 7.37° (P < .05). Partial tears created smaller openings that did not reach statistical significance (P > .05), with a maximum valgus opening of 2.30°.
Discussion
Several studies have suggested that an interaction exists between the olecranon and MUCL in stabilizing the elbow against valgus torques.2,4,5,10,18,22,33 Articular contact between the olecranon and the olecranon fossa significantly contributes to valgus stability as shown by An et al, who demonstrated that serial resections of the olecranon caused increasing valgus instability.2 Morrey and An determined that valgus stress in full elbow extension is equally divided between the MUCL, the capsule, and the articular surfaces.22 Clinically, King et al described “medial elbow-stress syndrome,” which included the triad of medial soft tissue insufficiency, posteromedial compartment impingement, and lateral compartment chondrosis.18 Wilson et al used the terminology “valgus extension overload” to describe the pathomechanics that associates medial elbow stress and isolated posteromedial impingement. 33 Development of posteromedial olecranon osteophytes has been found in overhead-throwing athletes with valgus extension overload.5,8 In patients with MUCL repairs or reconstructions who have had multiple operations, the rates of previous posteromedial osteophyte excision have varied from 36%7 to 44%10 to 86%.31 The present study confirmed the relationship between the MUCL and the posteromedial articulation by demonstrating altered contact within the posteromedial compartment of the elbow with increasing MUCL insufficiency; statistically significant changes were found with full tears at 30° of elbow flexion for area and pressure measurements. Contact area decreased and pressure increased with increasing MUCL insufficiency for all ligament and loading conditions for each
elbow flexion angle.
The nonoperative treatment for valgus extension overload injuries includes rest, anti-inflammatory medicine, correction of improper throwing mechanics, and a rehabilitation program to strengthen and coordinate the muscles acting across the elbow. If nonoperative treatment fails, decompression of the posterior compartment is indicated. Wilson et al have described a biplane osteotomy in which approximately 1.0 cm of the olecranon tip was excised, followed by a portion of the posteromedial olecranon.33 Andrews and Timmerman showed that following resection of elbow posteromedial osteophytes in baseball pitchers, as many as 25% required MUCL reconstruction.5 This clinical observation suggests that an interaction exists between the olecranon and the MUCL in maintaining valgus stability with overhead throwing. It has been hypothesized that olecranon resection places increased demand on the MUCL, which then leads to MUCL failure. Andrews et al, however, studied the effect of olecranon osteotomy on MUCL strain and found no statistically significant relationship. 4 Alternatively, the present study suggests that existing MUCL injury may lead to olecranon osteophytosis from contact alterations within the posteromedial elbow. Surgical treatment of olecranon resections may thereby unmask an already injured MUCL.
Several limitations exist with our experimental method that should be considered when interpreting the results. The valgus torque generated across the elbow from overhead throwing is the result of tremendous dynamic acceleration forces. This cadaveric study simulated valgus torque with a static load, which did not replicate the dynamic nature of throwing and the shear forces that occur. It is likely that shear forces also contribute to the pathology of olecranon osteophytes, which was not measured. However, the results of this study demonstrated gross contact alterations with MUCL insufficiency.
The testing method limited the number of flexion angles tested to 30° and 90° and not the entire arc of elbow flexionextension. Numerous reports have used 30° of elbow flexion for manual valgus instability testing as this unlocks the bony configuration of the elbow.10,25,32 Callaway et al showed that clinical testing for complete tears of the anterior bundle should be performed at 90° of elbow flexion.9 Other studies have shown that elbow flexion angles between 60° and 75° are optimal for revealing valgus instability.12,29,32 In the current study, 90° and 30° of elbow flexion were chosen to represent the late-cocking/acceleration (relative flexion) and deceleration (relative extension) phases of pitching, respectively. Our results confirm the pathomechanics of valgus extension overload,18,33 with significant changes in contact found in relative elbow extension (Table 3). During the deceleration phase of throwing, posteromedial olecranon osteophytosis may develop from repeated compression of the olecranon into the posteromedial trochlea if the deceleration is not well controlled by dynamic forces.8
Dynamic muscle forces were not evaluated in this study and may influence posteromedial elbow contact by affecting valgus stability. Morrey et al were the first to introduce the role of dynamic stability into an elbow valgus instability model by examining ulnohumeral joint compressive forces with biceps, brachialis, and triceps muscle simulations.24 Davidson et al have shown anatomically that the flexor carpi ulnaris is ideally positioned to assist the MUCL.11 The hypothesis that the flexor-pronator mass can help to reverse the increased valgus angle, and in turn the posteromedial contact alterations caused by MUCL insufficiency, requires a separate study. Several other studies have suggested that supination7,15 and pronation6,12 may influence valgus instability in overhead throwers. The present study design attempted to isolate the effect of MUCL insufficiency on posteromedial contact. The effects of pronation and supination as well as dynamic muscle loading on posteromedial elbow contact deserve further study.
The present study did not quantify the exact location of the contact alterations that occurred with MUCL insufficiency. The contact area was defined by the pressuresensitive film that was prepared in a standardized fashion to match the posteromedial trochlear curvature. As shown in Figure 2, the contact area reaches the lateral edge of the film, beyond which lies the lateral trochlea, an area not clinically involved in the development of posteromedial osteophytes. Measuring contact over the entire ulnohumeral joint complex curvature risks creating significant film artifact, as the flat pressure-sensitive film does not easily conform to highly curved surfaces. However, the area defined by the contoured pressure-sensitive film does capture the pathologic area found in the posteromedial elbow where osteophytes develop.
King et al highlighted the triad of MUCL strain, posteromedial elbow impingement, and radiocapitellar osteochondrosis in overhead-throwing pitchers.18 The lateral compartment provides a bony restraint that the current study did not measure with respect to contact area and pressure. The focus of the current study was to examine the common clinical problem of posteromedial elbow osteophytes. Future studies may address contact alterations in the radiocapitellar joint.
Our results suggest that MUCL insufficiency is a primary pathologic component in the development of posteromedial osteophytes. Avoiding increased demands on the MUCL in patients with posterior osteophytes is critical because these patients may have either existing laxity or MUCL insufficiency that contributes to the development of symptomatic posterior impingement. Treating bony impingement with osteotomy may convert an asymptomatic medially lax elbow into an unstable painful elbow. Alternatively, the MUCL may be insufficient but clinically stable secondary to osteophyte formation. Therefore, to assess the degree of MUCL stability, we recommend thorough evaluation of the MUCL when patients present with posteromedial elbow pain.
References
- Ahmad CS, Lee TQ, ElAttrache NS. Biomechanical evaluation of a new ulnar collateral ligament reconstruction technique with interference screw fixation. Am J Sports Med. 2003;31:1-6.
- An KN, Morrey BF, Chao EY. The effect of partial removal of proximal ulna on elbow constraint. Clin Orthop. 1986;209:270-279.
- Andrews JR. Bony injuries about the elbow in the throwing athlete. Instr Course Lect. 1985;34:323-331.
- Andrews JR, Heggland EJH, Fleisig GS, Zheng N. Relationship of ulnar collateral ligament strain to amount of medial olecranon osteotomy. Am J Sports Med. 2001;29:716-721.
- Andrews JR, Timmerman LA. Outcome of elbow surgery in professional baseball players. Am J Sports Med. 1995;23:407-413.
- Armstrong AD, Dunning CE, Faber KJ, Johnson JA, King GJW. Single-strand ligament reconstruction of the medial collateral ligament restores valgus elbow stability. J Shoulder Elbow Surg. 2002;11:65-71.
- Azar FM, Andrews JR, Wilk KE, Groh D. Operative treatment of ulnar collateral ligament injuries of the elbow in athletes. Am J Sports Med. 2000;28:16-23.
- Bennett JB, Green MS, Tullos HS. Surgical management of chronic medial elbow instability. Clin Orthop. 1992;278:62-68.
- Callaway GH, Field LD, Deng XH, et al. Biomechanical evaluation of the medial collateral ligament of the elbow. J Bone Joint Surg Am. 1997;79:1223-1231.
- Conway JE, Jobe FW, Glousman RE, Pink M. Medial instability of the elbow in throwing athletes. J Bone Joint Surg Am. 1992;74:67-83.
- Davidson PA, Pink M, Perry J, Jobe FW. Functional anatomy of the flexor pronator muscle group in relation to the medial
collateral ligament of the elbow. Am J Sports Med. 1995;23:245-250. - Field LD, Altchek DW. Evaluation of the arthroscopic valgus instability test of the elbow. Am J Sports Med. 1996;24:177-181.
- Fleisig GS, Barrentine SW, Escamilla RF, Andrews JR. Biomechanics of overhand throwing with implications for injuries. Sports Med. 1996;21:421-437.
- Fleisig GS, Barrentine SW, Zheng N, Escamilla RF, Andrews JR. Kinematic and kinetic comparison of baseball pitching among various levels of development. J Biomech. 1999;32:1371-1375.
- Fleisig GS, Escamilla RF. Biomechanics of the elbow in the throwing athlete. Oper Tech Sports Med. 1996;4:62-68.
- Hechtman KS, Tjin-A-Tsoi EW, Zvijac JE, Uribe JW, Latta LL. Biomechanics of a less invasive procedure for reconstruction of the ulnar collateral ligament of the elbow. Am J Sports Med. 1998;26: 620-624.
- Jobe FW, Stark H, Lombardo SJ. Reconstruction of the ulnar collateral ligament in athletes. J Bone Joint Surg Am. 1986;68:1158- 1163.
- King JW, Brelsford HJ, Tullos HS. Analysis of the pitching arm of the professional baseball pitcher. Clin Orthop. 1969;67:116-123.
- Kuroda S, Sakamaki K. Ulnar collateral ligament tears of the elbow joint. Clin Orthop. 1986;208:266-271.
- Merz B, Eckstein F, Hillebrand S, Putz R. Mechanical implications of humero-ulnar incongruity: finite element analysis and experiment. J Biomech. 1997;30:713-721.
- Morrey BF. Applied anatomy and biomechanics of the elbow joint. Instr Course Lect. 1986;35:59-68.
- Morrey BF, An KN. Articular and ligamentous contributions to the stability of the elbow joint. Am J Sports Med. 1983;11:315-319.
- Morrey BF, An KN. Functional anatomy of the ligaments of the elbow. Clin Orthop. 1985;201:84-90.
- Morrey BF, Tanaka S, An KN. Valgus stability of the elbow: a definition of primary and secondary constraints. Clin Orthop. 1991;265:187-195.
- O’Driscoll SW, Bell DF, Morrey BF. Posterolateral rotatory instability of the elbow. J Bone Joint Surg Am. 1991;73:440-446.
- Pappas AM, Zawacki RM, Sullivan TJ. Biomechanics of baseball pitching: a preliminary report. Am J Sports Med. 1985;13:216-222.
- Remia LF, Ravalin RV, Lemly KS, McGarry MH, Kvitne RS, Lee TQ. Biomechanical evaluation of multidirectional glenohumeral instability and repair. Clin Orthop. 2003;416:225-236.
- Sekaran SV, Hull ML, Howell SM. Nonanatomic location of the posterior horn of a medial meniscal autograft implanted in a cadaveric knee adversely affects the pressure distribution on the tibial plateau. Am J Sports Med. 2002;30:74-82.
- Sojbjerg JO, Ovesen J, Nielsen S. Experimental elbow instability after transection of the medial collateral ligament. Clin Orthop. 1987;218:186-190.
- Stormont TJ, An KN, Morrey BF, Chao EY. Elbow joint contact study: comparison of techniques. J Biomech. 1985;18:329-336.
- Thompson WH, Jobe FW, Yocum LA, Pink MM. Ulnar collateral ligament reconstruction in athletes: muscle-splitting approach without transposition of the ulnar nerve. J Shoulder Elbow Surg. 2001;10: 152-157.
- Timmerman LA, Schwartz ML, Andrews JR. Preoperative evaluation of the ulnar collateral ligament by magnetic resonance imaging and computed tomography arthrography: evaluation in 25 baseball players with surgical confirmation. Am J Sports Med. 1994;22:26-32.
- Wilson FD, Andrews JR, Blackburn TA, McCluskey G. Valgus extension overload in the pitching elbow. Am J Sports Med. 1983;11:83-87.