Ben H. Nelson1, Donald D. Anderson2, Richard A. Brand1i2 and Thomas D. Brown1,2
Full thickness osteochondral defects 6 mm in diameter were created in the weightbearing regions of the femoral condyles in 5 adult mongrel dogs to study the contact pressure changes accompanying healing. Adigital imaging technique employing Fuji Prescale film mapped contact pressures following 1 1 months of healing for comparison with contralateral normal knees, and knees with freshly made defects. Although all defects healed uneventfully with subchondral plate reconstitution and with growth restoration of the articular surface, the repair soft tissue appeared histologically to be primarily fibrous tissue, with varying degrees of a fibrocartilaginous component. The mean and peak stresses about fresh defects were not appreciably different from those about healed defects. Neither were there substantial differences in the total cartilage area making contact, except when very low loads were applied. The results suggest that the repair tissue is of poor mechanical quality, and does not contribute appreciably to weight bearing. The cartilage adjacent to the defect did notexperience high stresses; neither gross nor light microscopic evidence of degeneration appeared at 1 I months. If degeneration does occur following such defects, our data suggest that it is not because of elevated contact stresses.
The recent availability of pressure-sensitive film plus the development of optical scanning techniques for high-resolution analysis of the films has made it possible to determine local contact pressures on articular surfaces (Fukubayashi and Kurosawa 1980, Kimizuka et al. 1980, Hehne et al. 198 1, Dorenberg 1983, Tarret al. 1985, Brown et al. in press). We report on alterations in local joint contact pressures and contact areas produced by defects in the dog femoral condyle, both acutely and after healing.
Material and methods
Five conditioned adult mongrel dogs weighing 21 to 25 kg were anesthetized with barbiturate. They underwent median parapatellar arthrotomies, three bilateral and two unilateral (i.e., eight knees, 16 defects). We drilled osteochondral defects 6 mm in diameter in the anterior weight-bearing portions of both the medial and the lateral femoral condyles. The defects were made in that portion of the femur that contacts the tibia during stance phase, i.e., 3Oo-4Oo of flexion (Adrian et al. 1966). Postoperatively, the dogs were allowed free activity. After 47 (42-54) weeks, the animals were killed and their knees harvested.
After removal of extracapsular tissue, the femurs and tibias were potted in screw-reinforced dentalgrade polymethylmethacrylate (PMMA). The patellar tendon and patella were then reflected and the capsule was removed, including collateral ligaments, but sparing menisci, meniscotibial andcruciate ligaments. After India-ink staining (Meachim and Stockwell 1979) and gross photographs, the knees were mounted in a materials testing machine. The tibial mounting allowed the joint to seek its most stable position when loaded in compression, by incorporating semiconstrained rotation and limited varus-valgus freedom. The femur was fixed rigidly to the load cell of the testing machine. The amount of knee flexion was adjustable to allow positioning of the defect in the joint contact area. No attempt was made to load both compartments equally because medial and lateral defects did not usually fall within their respective contact patches at the same degree of flexion. Instead, the knees were placed in slight varus (medial compartment) or valgus (lateral compartment) angulation to ensure defect centering within the individual contact regions. This loading technique introduced variability in the load transmitted across the measured compartment; that is, of the applied 300 N load, most would be transmitted by the compartment being studied, but a variable amount would also be transmitted by the other compartment.
Contact recordings were made by placing cellophane- wrapped Fuji Prescale film between joint surfaces and applying a load of 300 N (0.1 second to achieve full load, load held for 0.2 seconds, and 0.1 second to release). Two film grades were used, so as to obtain two ranges of sensitivity: the low pressure film accurately measured 2 to 7, and medium pressure film 5 to 20 MPa. In four condyles, pressure recordings were unsatisfactory owing to the placement of the chronic defects, i.e., only a small amount of the defect and surrounding area was included in the pressure tracing. This left a total of 22 determinations in 12 condyles. Similar pressure measurements were made on three of four unoperated condyles and on four with “acute” defects.
Using the technique of Singerman et al. (1987), the staining intensity was digitized and integrated using an Eye-Com II scanner, allowing the calculation of contact area, total load, and peak and mean contact pressures. The scanner divided a given view field into a large number of pixels. The magnification of the scanner is variable; for this experiment, resolutions corresponding to 25 to 32 pixels per square millimeter were used. Each pixel was automatically assigned one of 256 integers, representing a “shade of gray” derived from its film-staining intensity. The loading pressure experienced by a given pixel was computationally determined by comparing its shade of gray to those of identical film stained under known loading conditions. The number of pixels stained mutiplied by the pixel area yielded the total contact area. Incremental load histograms were calculated by multiplying the number of pixels in a given range of staining intensities (shades of gray) by the mean pressure in that range, as determined from the calibration curve for that knee. The sum of these incremental loads from the various ranges approximates the total applied load. Previous studies have demonstrated recovery of approximately 85 percent of applied load using this technique (Loren et al. 1983, Tarr et al. 1985, Brown et al. 1988).
Following the loading experiments, six of the eight femurs and tibias were decalcified, sectioned, and stained with hematoxylin and eosin (HLE) and with Safranin 0 and examined microscopically.
Results
All the wounds healed uneventfully. After 10 to 12 months, none of the dogs limped, although 1 dog had a 25Oflexion contracture in one knee. The defects were readily apparent (Figure 1) after 10 to 12 months, with fibrillated tissue projecting above the surface of the adjacent cartilage. Histologic studies showed reconstitution of the subchondral plate in all the chronic defects. Variable amount of fibrocartilage filled the defects, the surface of which was was usually fibrillated. Whereas the defect replacement
tissue was cartilaginous, virtually no Safranin 0 staining occurred in that tissue, indicating the nearly complete absence of proteoglycans. The cartilage immediately adjacent to and opposite the defects (on the tibia) was normal, both on H&E and Safranin 0 staining.
The loads calculated from integrating the measured compartment pressures varied from 49 to 347 N. Although we attempted to load primarily the compartment of interest, some of the 300 N load was transmitted by the other compartment. The single case of recovery higher than the applied load (i.e., the 347 N load) demonstrates that the process of integrating the pressures is accurate only within the range of 15 percent.
The calculated contact area ranged from 40 to 166 mm2, and increased with applied load. These contact area determinations did not include the approximately 28mm2 area of the defects. Mean pressure values (calculated load divided by contact area) ranged from l .06 to 2.64 MPa, whereas peak pressures ranged from 2.5 to 12.3 MPa. Mean and peak pressures increased with applied load, both in acute and chronic defects (Figure 2). Correlation coefficients for pressure versus load ranged from 0.35 to 0.79 for the mean pressure and from 0.22 to 0.99 for the peak pressure.
Load-versus-area plots demonstrated similar contact area curves for medial and lateral condyles with chronic defects (Figure 3). Combined, their correlation coefficient with applied load was 0.93. The contact area curves for knees without defects and those with acutely formed defects were nearly superimposable and were slightly beneath (about 12 mm2) the chronic defect curve. Correlation coefficients were 0.99 for no defect and 0.97 for the acute defect.
Discussion
Numerous morphologic and biochemical studies have documented the inability of superficial cartilage defects to undergo repair (Calandruccio and Gilmer 1962,Campbell1969, Convery et al. 1972, DePalmaet al. 1966, Mankin 1974, Meachim 1963, Meachirn and Roberts 1971, Mitchell and Shephard 1976 and 1980, Salteret al. 1980). However, if the defect involves subchondral bone, acutely formed granulation tissue is followed by hyaline-appearing cartilage or fibrous and fibrocartilaginous tissue (Mankin 1982, Buckwalter 1983). Although the long-term effectiveness of this repair process has been poorly documented, it forms the basis for the clinical drilling of both acute and chronic osteochondral defects (Rand 1985). A recent study by Whipple et al. (1986) questions the ability of the fibrocartilaginous replacement tissue to substitute for hyaline cartilage.
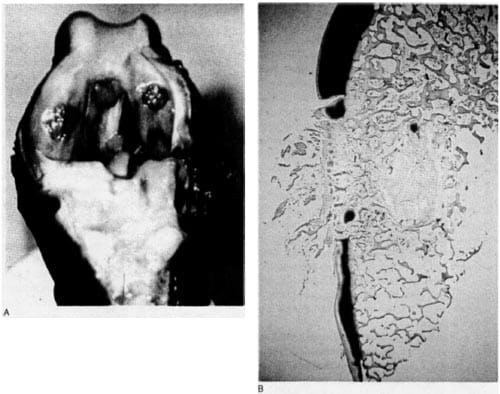
Figure 1. Canine knee with 6-mm chronic defects. A. Fibrocartiiaginous tissue fills the defects. B. Safranin 0 preparation of a 6-mm chronic defect, demonstrating nearly complete absence of proteoglycans in defect repair tissue with normal staining adjacent to lesion. The subchondral bone plate has been restored by a new “plate.”
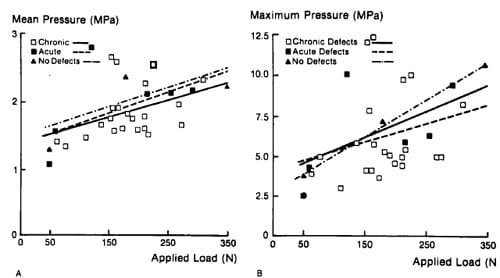
Figure 2. Applied load (P) versus mean pressure (Pavg) and maximum pressure (Pmax). Linear regressions and correlation coefficients for the chronic, acute, and no-defect groups, respectively, were above.
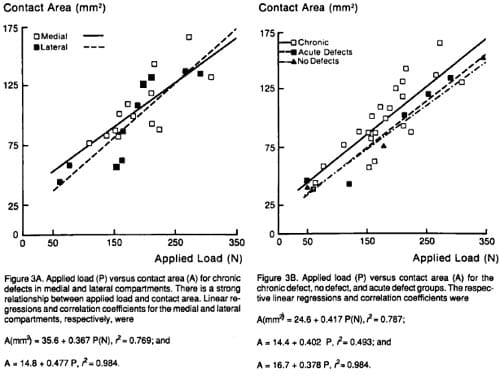
Histologic observations of the joints in the present model were consistent with the results of previous studies (Furukawa et al. 1980, Meachim and Roberts 1971, Mitchell and Shephard 1976). The tissue that filled the chronic osteochondral defects was morphologically fibrocartilaginous, but contained very little proteoglycan. However, the question of whetherrepair tissue is present is distinct from whether and how this repair tissue functions. The degree to which repair tissue functions may be reflected in its ability to distribute load.
The 300 N loads (roughly one times body weight) used in our experiment were subphysiologic (Alexander 1974). and may be viewed analogously to the subphysiologic (1,000-2,000 N) loads previously used for bicondylar contact studies of the human knee (Ahrned and Burke 1983, Fukubayashi and Kurosawa 1980, Kimizuka et al. 1980). Contact areas in the human knee appear to saturate slightly above one times body weight (Brown and Shaw 1984). Assuming that the contact area of the dog knee approaches saturation at joint loads slightly above one times body weight as in the human, one would expect the local pressures to increase approximately linearly with further increases in joint loads beyond 300 N. It seems unlikely, therefore, that higher loads would eventually load the new fibrocartilage in the defect.
Intuitively, acute defects would decrease available surface area; but since the load-versus-area curves without and with acute defects were virtually identical, peripheral contact area was recruited rather surprisingly in an amount approximately equal to the defect area. Presumably, this is owing to the compliance of the cartilage. Knees with chronic defects had slightly increasedcontact areas at any given load when compared with acutely created defects or with those knees without defects. However, we doubt that such a small increase has physiologic significance. None of this increase was related to load of repair tissue, because in no case did we see Fuji stain in the region of the defects. Thus, the area transmitting detectable load does not seem to be dependent upon the presence of defects of this size or whether or how they heal. Neither mean nor peak pressures were detectably affected by the presence of acute or chronic defects, but both increased slightly with applied load in the load range tested.
Our results suggest that articular cartilage of adult dog knees can accommodate moderate incongruities without major peak pressure alterations, and without obvious morphologic consequences. Assuming that therelationshipbetween peakpressure and joint load is linear for higher loads than those used here, our defects would still not produce peak pressures above the 25 MPa maximum tolerable limit suggested by Repo and Finlay (1977). This is consistent with our observation of no degenerative changes, although we cannot be certain whether degenerative changes would have become apparent with a much longer period of observation. However, the fact that so-called degenerative changes appear in less than 1 year in dogs following sectioning of the anterior cruciate ligament (Pelletier et al. 1985) suggests that our period of observation would have been long enough to see some earlychanges. The findings that these defects neither reduced contact areas nor increased mean and peak contact pressures suggest that articular surface incongruities do not necessarily lead to rapid degeneration because of high pressures.
References
- Adrian M J, Roy WE, Karpovich P V. Normal gait of the dog: an electrogoniometric study. Am J Vet Res 1966;27( 1 16): 90-5.
- Ahmed A M, Burke D L. In vitro measurement of static pressure distribution in synovial joints Part I: Tibia1 surface of the knee. J Biomech Eng 1983;105(3):216-25.
- Alexander R M. The mechanics of jumping by a dog (Canis Familiaris). J Zool Proc Zool Soc Lond 1974;173(4): 549-73.
- Brown T D, Anderson D D, Nepola J V, Singerman R J, Pedersen D R, Brand R A. Contact stress aberrations following imprecise reduction of simple tibia1 plateau fractures. J Orthop Res. In press.
- Brown T D, Shaw D T. In vitro contact stress distribution on the femoral condyles. J Onhop Res 1984;2(2):190-9.
- Buckwalter J A. Artic
ular cartilage. Instr Course Lect 1983; 32349-70. - Calandruccio R A, Gilmer W S. Proliferation, regeneration, and repair of articular cartilage of immature animals. J Bone Joint Surg (Am) 1962;44:431-55.
- Campbell C J. The healing of cartilage defects. Clin Orthop 1969;64:45-63.
- Convery F R, Akeson W H, Keown G H. The repair of large osteochondral defects. An experimental study in horses. Clin Orthop 1972;(82):253-62.
- DePalma A F, McKeever C D, Subin D K. Process of repair of articular cartilage demonstrated by histology and autoradiography with tritiated thymidine. Clin Orthop 1966;(48):229-42.
- Dorenberg K 0. Kontaktflachen und anatomische Gelenkflachen des oberen Sprunggelenks. Methoden zur Bestimmung der Flachengr6sse und Falldarstellungen. Morphol
- Fukubayashi T, Kurosawa H. The contact area and pressure distribution pattern of the knee. A study of normal and osteoarthrotic knee joints. Acta Orthop Scand 1980; 51(6):871-9.
- Furukawa T, Eyre D.R., Koide S, Glimcher, M J. Biochemical studies on repair cartilage resurfacing experimental defects in the rabbit knee. J Bone Joint Surg (Am) 1980 62( 1):79-89.
- Hehne H J, Ficker E, Jantz W, Mahr D, Schopf H J. Eine neue Methode zur Ermittlung lastabhhgiger Druck und Kontaktverlaufe an Gelenkflkhen. Morphol Med 198 1 ; l(2):95-106.
- KimizukaM,KurosawaH,FukubayashiT. Loadbearingpattern of the ankle joint. Contact area and pressure distribution. Arch Orthop Trauma Surg 1980;96(1):45-9.
- Lorenz M, Patwardhan A, Vanderby R Jr. Load bearing characteristics of lumbar facets in normal and surgically altered spinal segments. Spine 1983;8(2): 122-30.
- Mankin H J. The reaction of articular cartilage to injury and osteoarthritis. Part I. N Engl J Med 1974;291(24): 1285-92.
- Mankin H J. The reaction of articular cartilage to injury and osteoarthritis. Part II. N Engl J Med 1974;291(25):1335-40.
- MankinHJ.Currentconceptsreview.Theresponseof articularcartilage to mechanical injury. J Bone Joint Surg (Am) 1982;64:460-6.
- Mankin H J, Dorfman H, Lippiello L, Zarins A. Biochemical and metabolic abnormalities in articular cartilage from osteo- arthritic human hips. II. Correlation of morphology with biochemical and metabolic data. J Bone Joint Surg (Am) 197 1;53(3):523-37.
- Meachim G. The effect of scarification on articular cartilage in the rabbit. J Bone Joint Surg (Br) 1963;45: 15061.
- Meachim G, Roberts C. Repair of the joint surface from subarticular tissue in the rabbit knee. J Anat 1971;109(2):
- Meachim G, Stockwell R A. In: Adult Articular Cartilage (Ed. Freeman M A R.). 2nd ed. Pitman Medical, Tunbridge Wells 1979:524.
- Mitchell N, ShepardN. The resurfacing of adult rabbit articular cartilage by multiple perforations through the subchondral bone. J Bone Joint Surg (Am) 1976;58(2):230-3.
- Mitchell N, Shepard N. Healing of articularcartilage in intraarticularfractures in rabbits. JBone Joint Surg(Arn) 1980;62(4):628-34.
- Pelletier J P, Martel-Pelletier J, Ghandur-Mnaymneh L, Howell D S. Woessner J F Jr. Role of synovial membrane inflammation in cartilage matrix breakdown in the Pond Nuki dog model of osteoarthritis. Arthritis Rheum 1985;28(5):554-61.
- Rand J A. Arthroscopy and articular cartilage defects. Conternporthop 1985;11:13.
- Repo R U, Finlay J B. Survival of articularcartilage aftercontrolled impact. J Bone Joint Surg (Am) 1977;59(8):106&76.
- Salter R B, Simmonds D F, Malcolm B W, Rumble E J, Mac- Michael D, Clements N D. The biological effect of continuous passive motionon the healing of full thickness defects in articularcartilage. An experimental investigation in the rabbit. J Bone Joint Surg (Am) 1980;62(8): 1232-5 1.
- Singerman R J, Pedersen D R, Brown T D. Quantitation of Fuji Presensorpressures using digital image scanning. Exp Mech 1987;27:9%105.
- TarrRR,ResnickCT, WagnerKS, SarmientoA.Changesin tibiotalar joint contact areas following experimentally induced tibia1 angular deformities. Clin Orthop 1985; (199):72-80.
- Whipple R R,Gibb M C,Lai W M, Mow V C, Mak A F, Wirth C R. Biphasic properties of repaired cartilage at the articular surface. Trans Orthop Res Soc 1986;10:340.